“For you are dust, and to dust you shall return” (Genesis 3:19)
How did life on Earth begin? Recent scientific advances suggest that all living beings are descended from a single gene that existed around 4 billion years ago. This gene produced two proteins that created the genetic code, the universal language of life that marks the separation from the inanimate world. The scientists who discovered these proteins, recognizing their central role at the dawn of existence, gave them familiar names: Adam and Eve.
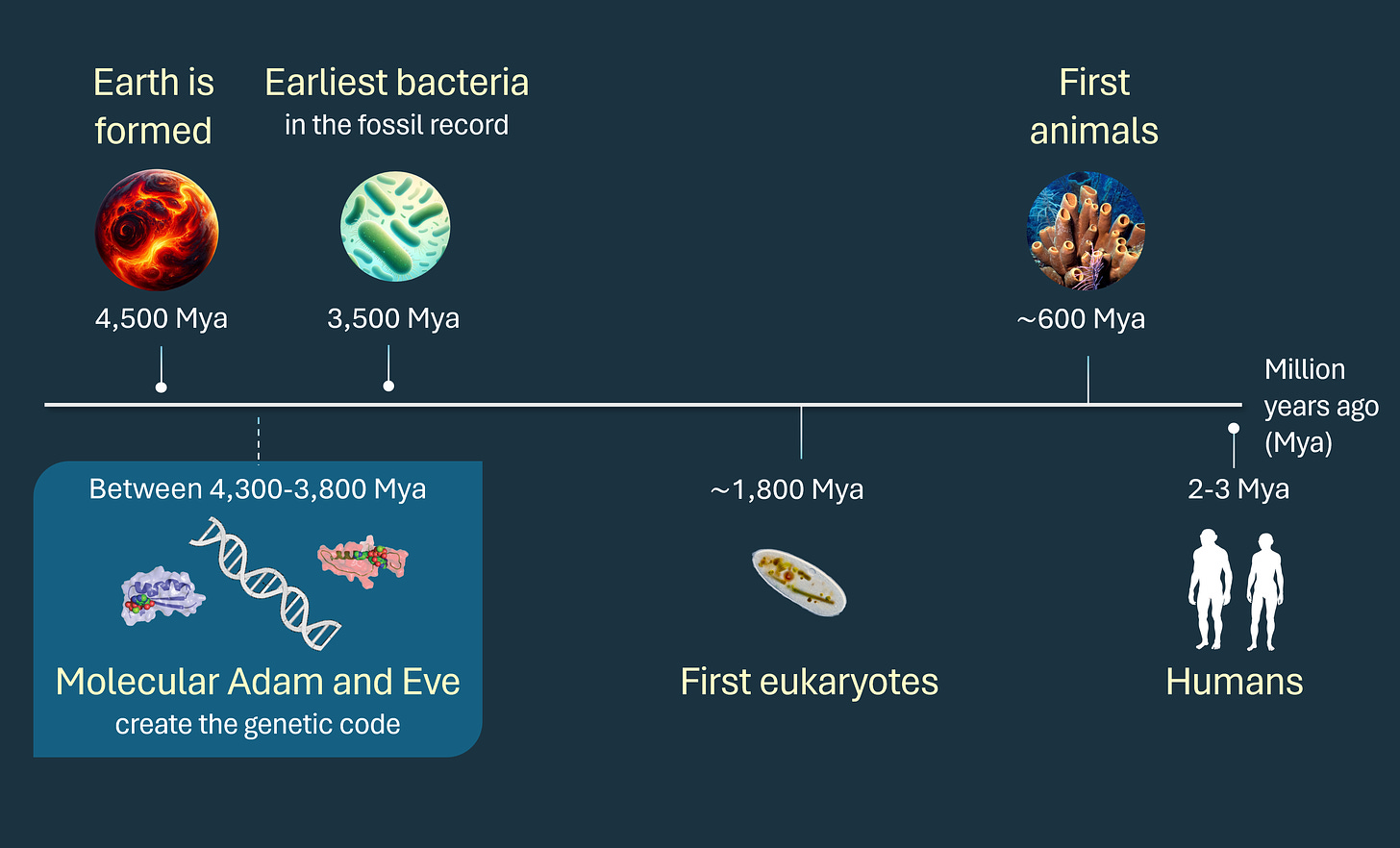
Darwin's Promise: A Path to the Origin
“God is dead. God remains dead. And we have killed him. How shall we console ourselves, the murderers of all murderers?”1 German philosopher Friedrich Nietzsche wrote these words a decade after a new scientific theory shook the prevailing religious dogma to its core. In his 1871 book The Descent of Man, English naturalist Charles Darwin openly defied the Biblical narrative of Adam and Eve: human beings could not have been created directly by God in the Garden of Eden, because they were the product of evolution and shared a common ancestor with apes2. In the context of the origin of life, Nietzsche’s statement was eerily accurate: Adam and Eve were dead, and Darwin was the executioner.
The void left by Adam and Eve’s demise was occupied by a tantalizing promise. Darwin’s theory established a familial link between all living organisms, which would mean that “probably all the organic beings which have ever lived on this Earth have descended from some one primordial form, into which life was first breathed."3 If the evolutionary tree could be traced back to its very root, such was the promise, the secret of the origin of life would be revealed.
Advances in molecular biology in the second half of the 20th century validated Darwin’s hypothesis. The common origin was beyond any reasonable doubt: all organisms without exception use the genetic code, a code so universal yet so complex that it is impossible to envision it emerging multiple times independently. To perform their essential functions, all cells need to make proteins that are encoded in DNA sequences; the genetic code is the language that translates the genetic information from the four-letter alphabet of DNA to the twenty-letter alphabet of proteins. During evolution, natural selection acts on small changes in the sequence of DNA that accumulate as they are passed from generation to generation. The genetic code, however, has remained almost unchanged since the first microbes began to populate the Earth. Species evolve, but the code is an immutable facilitator. Any attempt to decipher the origin of life must address this question: how did the code that enables everything else come to be?
The answer is present, like a living fossil, in every cell of every living creature on the planet. It remained encrypted until 1995, when pioneering scientists Sergei Rodin and Susumu Ohno followed Darwin's trail to its very end and found a singular ancestral gene simultaneously coding for two proteins in a perfectly complementary manner. Brilliant but esoteric, the Rodin-Ohno hypothesis faded into obscurity until the laboratory of Charles Carter, an obstinate American biochemist, demonstrated it experimentally two decades later. Biblical Adam and Eve had fallen, but at the end of the Darwinian path that replaced them lay an equally mystical and fascinating duo: molecular Adam and Eve, the two proteins that created the genetic code.
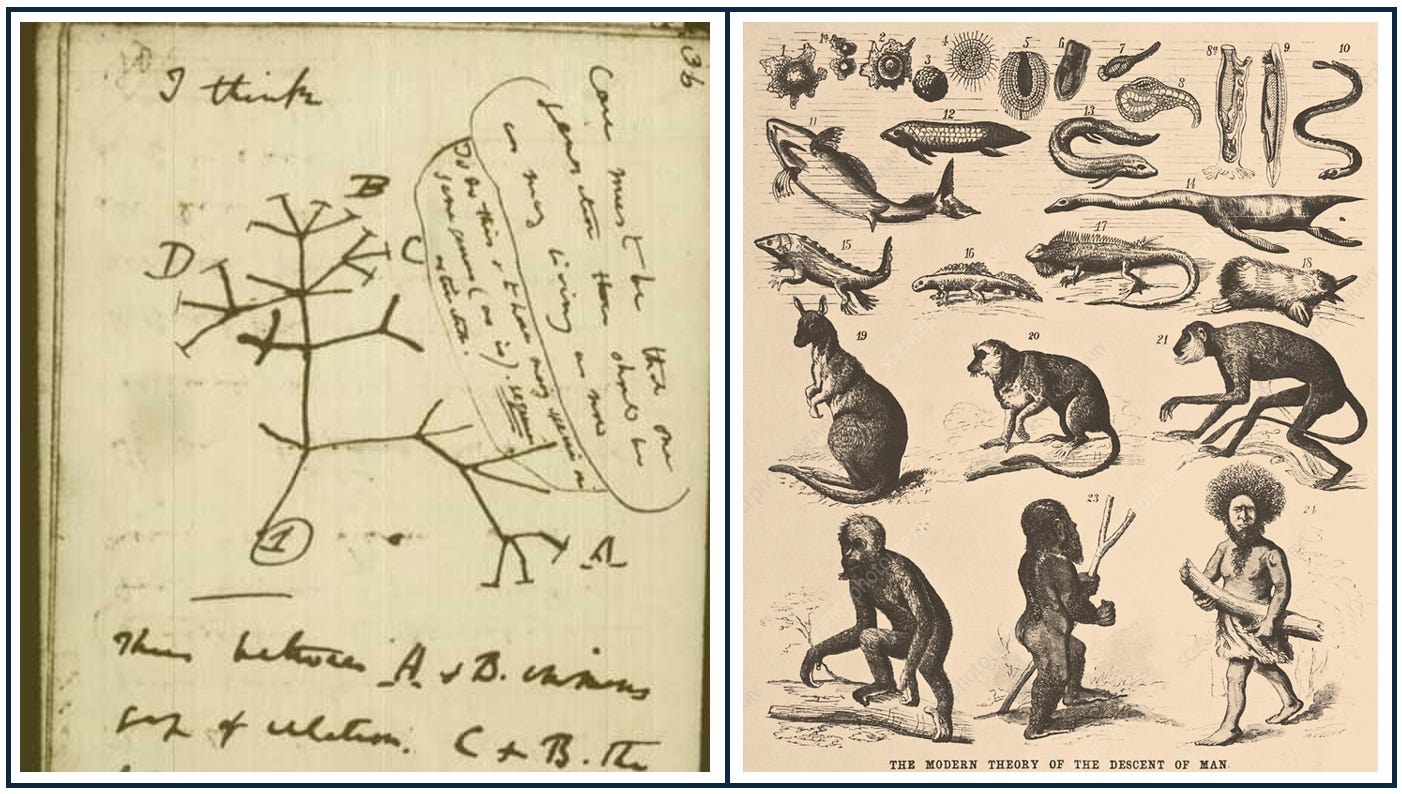
A Code to Rule Them All
Proteins are the most extraordinary molecules on Earth, perhaps even in the entire universe. They are the molecules that perform nearly all the vital functions of living beings: from generating energy and synthesizing organic matter to enabling movement and communication. Despite their seemingly simple structure—repetitive chains of twenty different amino acids—they are powerful molecular machines, capable of catalyzing a vast range of chemical reactions with exquisite speed and precision. The success of proteins lies in the fact that while their function depends on three-dimensional folds, the information to produce them is stored linearly in DNA molecules. The ease of DNA replication through the generation of a complementary strand pairs perfectly with the versatility of proteins to generate new functions as their sequence changes.
The partnership between DNA and proteins, with RNA serving as a crucial intermediary, is governed by the set of rules defined by the genetic code. It is a convoluted code without any seeming internal logic—a puzzle that stumped leading researchers Marshall Nirenberg and Har Gobind Khorana in the 1960s as they laboriously identified which of the 20 amino acids are specified by each of the 64 possible DNA base triplet combinations. Understanding the origin of the genetic code involves a paradox: the code is read and interpreted by proteins that are themselves encoded in it. The ribosome, the cellular machine responsible for protein synthesis, is made of a mixture of proteins and RNA. The translation from the alphabet of DNA bases to that of amino acids is carried out by a specialized group of proteins called aminoacyl-tRNA synthetases (hereafter referred to as synthetases for simplicity). These are the molecules that implement the code because they perform the critical task of reading base triplets and selecting the corresponding amino acids, which they then attach to transfer RNAs (tRNAs, adapter molecules) used during protein synthesis.
There are 20 different types of synthetases, one for each of the 20 amino acids that make up proteins. All living organisms must have at least one of each type, and each of these synthetases has a slightly different sequence that allows it to recognize its corresponding amino acid and tRNA. This specificity had to evolve over millions of years of Darwinian evolution. Yet the prerequisite for protein evolution is a preexisting code, creating an apparently unsolvable cycle akin to the chicken-or-egg- dilemma: what came first, the code or its interpreters?
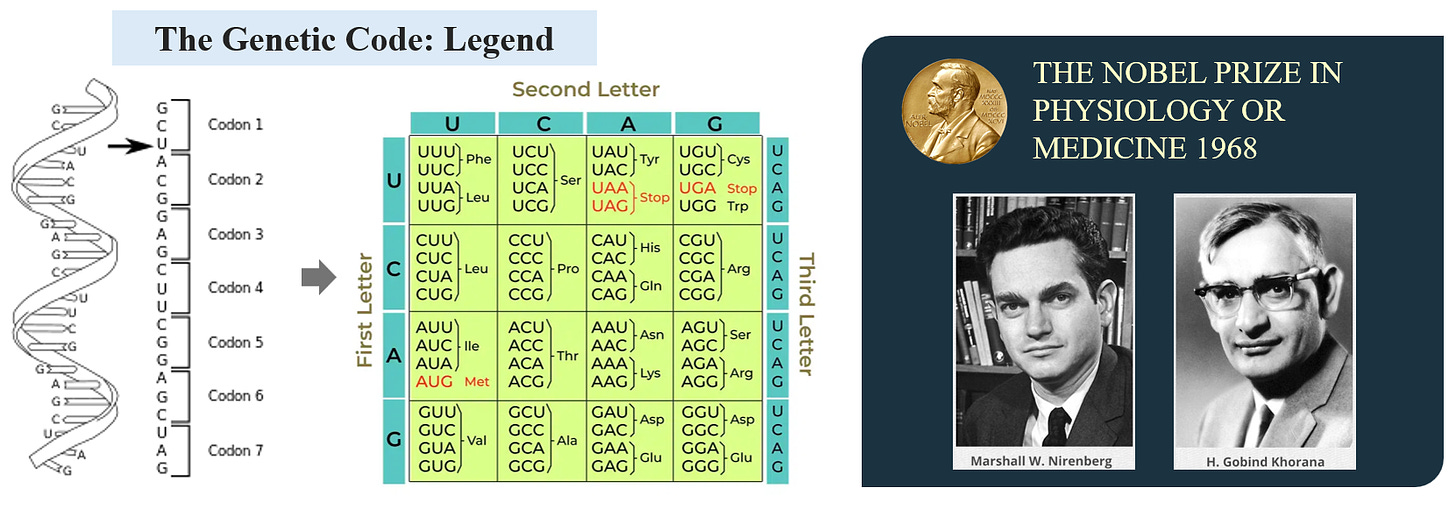
A Treasure Hunt to the Beginning of Time
In the early 1990s, Novosibirsk University Professor Sergei Rodin and his son Andrei enjoyed passing the long Russian winters unraveling biological puzzles. Molecular biology was in the midst of a long golden era, ignited by the invention of a groundbreaking DNA sequencing method in 1977 by English biochemist Fred Sanger. This technique, which helped uncover relationships among living organisms, was revolutionizing fields as diverse as human genetics, pathogen research, and the development of recombinant drugs.
Sergei and Andrei turned their attention to the study of tRNA, the adapter molecule that facilitates protein synthesis. Together, they uncovered evidence within current tRNA sequences of a primordial system, hinting at the early stages of the genetic code4. Their findings caught the attention of Japanese scientist Susumu Ohno, a renowned evolutionary biologist known for his contributions on gene duplication, who led a laboratory at the City of Hope Medical Center near Los Angeles. During a scientific conference in Moscow, Ohno persuaded Sergei Rodin to swap the cold of Siberia for the warmth of California and join him in tackling a central problem in the paradox of the origin of life: the role of aminoacyl-tRNA synthetases as the code interpreters.
Sergei Rodin and Susumu Ohno focused on trying to provide an explanation for a finding that baffled experts: the existence of two entirely different classes of synthetases. To understand this, consider that just as DNA sequence similarity between cousins indicates a recent common ancestor, so does protein sequence similarity suggest a common evolutionary precursor. For instance, humans see in three colors thanks to the action of three closely related opsin proteins, each adapted to detect a specific wavelength of light (blue, red, and green). The similarity in their sequences indicates that they evolved from a single ancestral opsin, like the one found in more primitive animals that perceive only one color.
In the case of synthetases, sequence analysis by various groups in the late 1980s revealed similarities among them, but with an important caveat: the 20 synthetases were divided into two separate classes, completely distinct in terms of sequence and three-dimensional structure56. The reason this was surprising is that proteins that catalyze very similar chemical reactions tend to be related to one another, indicating a single evolutionary origin. Even more intriguing, for the 20 amino acids that exist and make up proteins in all living organisms, scientists discovered a perfect symmetry: exactly half (10) are decoded by Class I synthetases, and the other half by Class II. This suggested that billions of years ago, the ancestors of Class I and Class II synthetases originated separately, each evolving over millions of years into 10 different protein families. Explaining how the function that implements the genetic code evolved once was challenging; explaining how it evolved twice seemed insurmountable.
Rodin and Ohno contended that the perfect symmetry between the two classes could not be a mere coincidence. Could it be hiding a fundamental property of the code, essential for its functioning? The two scientists embarked on a meticulous analysis of numerous synthetase sequences, looking for patterns that could establish some link between Class I and Class II and provide a clue on their evolutionary past. Despite exhaustive efforts, their search seemed destined for failure: the protein sequences of the two groups shared no discernible similarity. Shifting their focus from the proteins to the underlying DNA sequences that encode the two classes, however, led to a major turning point. Suddenly, a striking relationship emerged: in a mesmerizing pattern, the triplets coding for Class I synthetases tended to be complementary and in reverse order to those for Class II. Their statistical analysis yielded such a strong correlation that it all but ruled out any possibility of coincidence. The implications of this finding became immediately clear to the two researchers: going back in time, the only way such pattern could have emerged was through one single gene. A primordial gene that simultaneously encoded the ancestor of all Class I synthetases on one strand, and the ancestor of all Class II synthetases on the reverse complementary strand.
Rodin and Ohno’s breakthrough pointed to the moment in time where chemistry gave rise to biology. If their hypothesis was correct, the gene they postulated had created a language that would be the backbone of an explosion in complexity that has been growing exponentially for 4 billion years. They envisaged this gene to be made of RNA, because DNA is generally accepted to be a more recent evolutionary invention. Despite its transcendental significance, their discovery was largely overlooked. Unlike other fields like physics, purely theoretical studies in molecular biology are uncommon and are often met with skepticism by a scientific community used to the tangibility of wet lab data. Rodin and Ohno published their results in 1995 in the journal Origins of Life and Evolution of the Biosphere, a relatively niche publication7. Their article received scant recognition, reflected in the minimal number of citations from subsequent studies.
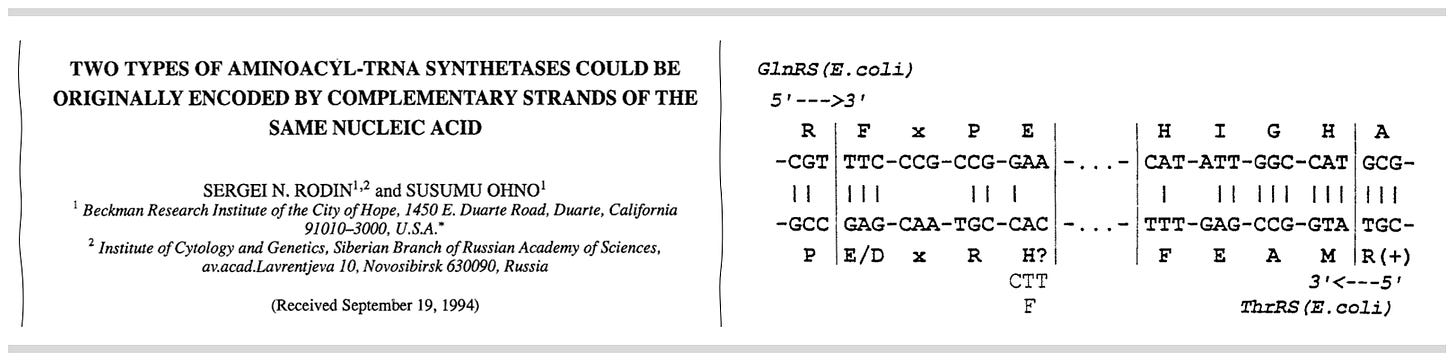
It was not until after the turn of the millennium that their work began to attract some attention. An article published in 2002 in Molecular Cell, a journal with a wide readership among molecular biologists, proclaimed that the Rosetta Stone validating the Rodin-Ohno hypothesis had been found8. The lead author was Charles Carter, a professor at the University of North Carolina who had spent his career studying three-dimensional structures of proteins with the question of the origin of life in mind. Carter’s bold assertion was grounded in a 1994 study he had recently stumbled upon: it reported that a species of freshwater mold contained a bidirectional gene that, for the first time seen in a living being, mirrored the concept proposed by Rodin and Ohno9. Specifically, in this particular mold gene, the DNA sequence coding for one protein in one strand is perfectly complementary to the DNA sequence coding for a second protein in the reverse strand. Furthermore, these two proteins are each distantly related to one of the two classes of tRNA synthetases: glutamate dehydrogenase to Class I, and Hsp70 to Class II. Carter reasoned that this had to be a living relic of the original Rodin and Ohno gene.
In the years that followed, Carter transformed his laboratory to test Rodin and Ohno's theory experimentally. His team carefully dissected the various domains within synthetases, determining which parts were evolutionarily older and which were more recent. As they went back in time, the symmetry between the two synthetase classes was consistently maintained: at each evolutionary stage, both classes shared similar sizes, mirror configurations (with the start of Class I corresponding to the end of Class II), and most importantly, an underlying DNA sequence with a high degree of complementarity10111213. The decisive breakthrough occurred in 2015, when, with the help of computational tools, the Carter lab constructed a candidate sequence for the primordial Rodin and Ohno gene: a segment of DNA that encoded two 46 amino acid-long proteins, one on each strand and in a perfectly complementary manner, that respectively contained the ancestral core of each of the synthetase classes. They synthesized the two proteins in the laboratory and, in a pivotal moment, demonstrated that both were biochemically active14.
These two proteins, emerging as mirror images from the same gene, form the foundation of all subsequent encoded proteins. Given their central role in the inception of the genetic code—perhaps the most critical moment in the origin of life—Carter named them Αδάμ and Εωε (Adam and Eve, in Greek characters)15. Their existence underscores a fundamental principle: a code can only arise when there are at least two options to choose from.
Through the pioneering biochemical experiments conducted by the Carter laboratory, it was revealed that molecular Adam and Eve exhibited distinct specificities towards different groups of amino acids. This specialization allowed them to carry out an initial, albeit rudimentary, production of proteins (themselves), marking a significant advance over random synthesis. Over millions of years of evolution, these two proteins each diversified into ten distinct forms. The fact that the symmetry was maintained between the two classes suggests that they were still part of bidirectional genes as they coevolved. The diversification process mirrored the complexity of the genetic code itself: the incorporation of new amino acids led to the addition of new codons, and at the same time allowed the synthetases to become more accurate at decoding genetic information. This cycle of coevolution between the code and its interpreters elegantly exemplifies how a code can be refined by the very entities it generates.
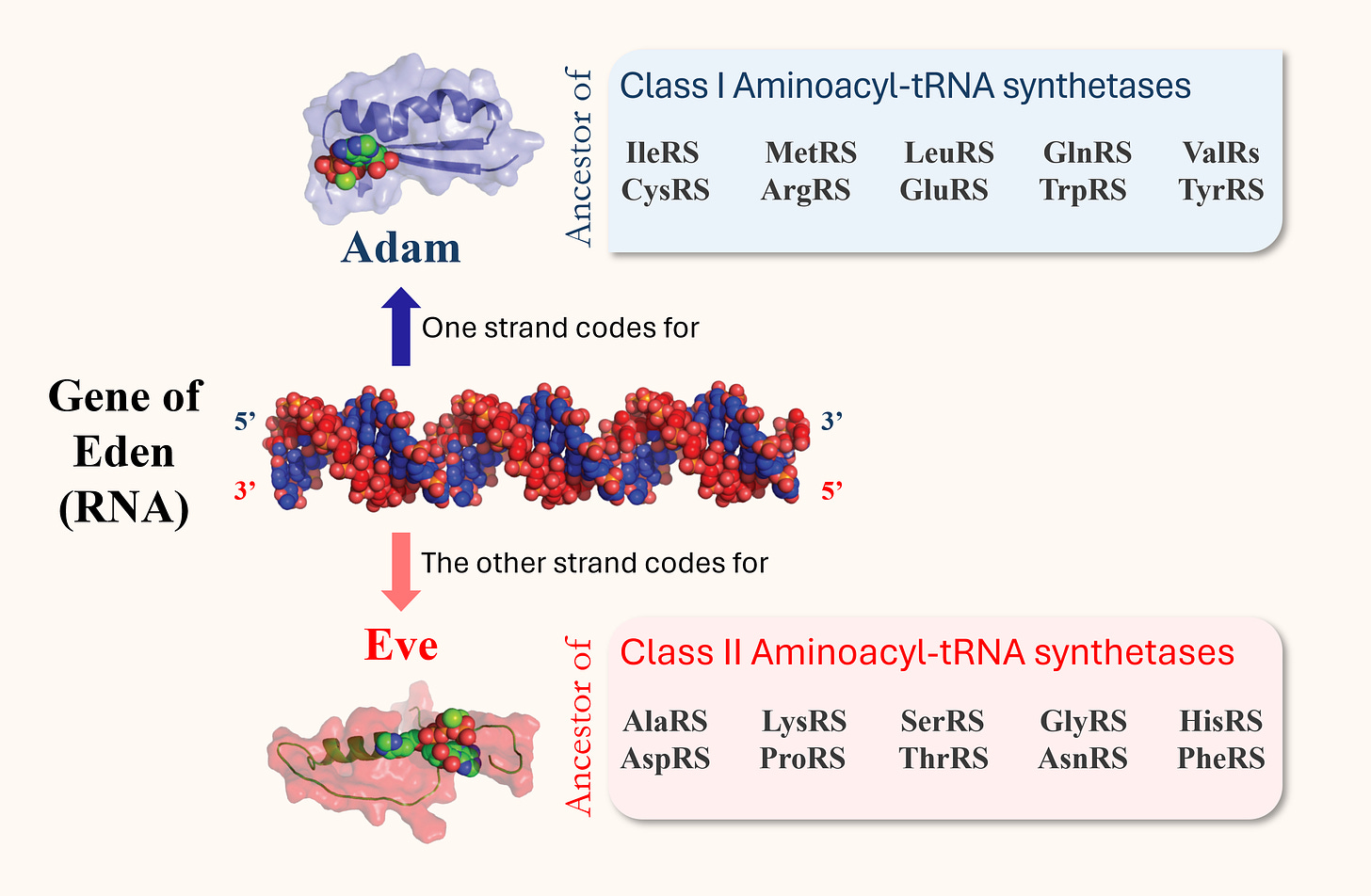
Adam and Eve Live On
Despite recent technological advances in chemistry and genetics, there is no universally accepted model for how life originated from inorganic matter. Fundamental questions about the location, energy source, and the identity of the first biological molecules to evolve remain controversial. Molecular Adam and Eve do not answer all these questions, but, like their Biblical counterparts, offer a snapshot of creation. In The Descent of Man, Darwin tacitly refuted Biblical Adam and Eve and proposed a hypothetical tree of life linking all living organisms to a single evolutionary origin. Today, we know that at the root of Darwin's tree are molecular Adam and Eve.
The existence of these two ancestral aminoacyl-tRNA synthetases offers a hardly intuitive explanation for life's origins, diverging from the simple elegance of the Neo-Darwinian model. The primordial gene—we can refer to it as the Gene of Eden— underpins the existence of a very rudimentary initial code, that became progressively more complex. The idea of a code recursively defining itself is hard to fully grasp. Yet the existence of a single ancestral gene provides an explanation to the genetic code's origin paradox: the only way to create a code whose interpreters are encoded by the code itself is through an iterative process in which code and interpreters emerge in tandem.
The discovery of molecular Adam and Eve remains far from the public spotlight, eluding broad recognition even within the scientific community. Perhaps its remote, almost metaphysical nature is not well aligned with the zeitgeist of the 21st century, centered on engineering advances. Or it could be the uncomfortable feeling that an important piece of the puzzle is still missing. Bridging the gap from the inanimate world of Earth's early days to the significant complexity of Adam and Eve is not a straightforward process; so much so that Sergei Rodin, the theory’s initiator, and Charles Carter, its validator, had opposing interpretations of what came immediately before. While Rodin believed in the existence of a pre-protein RNA world16, a widely held but unproven theory in the origin-of-life field, Carter contended that life started with coevolution between very short proteins and RNA sequences17. This discrepancy exposes the fact that Adam and Eve do not offer a self-evident explanation for how the Gene of Eden replicated, and reproduction is a key component required for Darwinian evolution. Despite these unresolved questions, the overwhelming evidence of the existence of Adam and Eve stands as a monumental discovery. They left an undeniable trace to the present day, visible through the phylogenetic tree of synthetases and their still recognizable specular configuration1819.
The Genesis myth about the creation of humans in the Garden of Eden emerged over 2,500 years ago in a small Eastern Mediterranean community. Over the centuries, it spread along with the three major Abrahamic religions —Judaism, Christianity, and Islam —becoming the predominant explanation for humanity’s beginnings throughout the world. The advent of Darwinism in the 19th century led to its decline, and religious precepts gradually gave way to scientific ones. With the discovery of the origin of the genetic code, however, Adam and Eve live on as a powerful metaphor of creation. They represent a unique moment in time in the distant past, at an undetermined location on planet Earth, where the duality of two perfectly complementary beings started the journey of existence.
This article was edited by Daniel Holoch
References
Nietzsche, F. The Gay Science. 1882
Darwin, C. The Descent of Man. 1871
Darwin, C. On the Origin of Species. 1859
Rodin, S., Ohno, S. & Rodin, A. Transfer RNAs with complementary anticodons: could they reflect early evolution of discriminative genetic code adaptors? Proc. Natl. Acad. Sci. U.S.A. 90, 4723–4727 (1993).
Eriani, G., Delarue, M., Poch, O., Gangloff, J. & Moras, D. Partition of tRNA synthetases into two classes based on mutually exclusive sets of sequence motifs. Nature 347, 203–206 (1990).
Cusack, S., Berthet-Colominas, C., Härtlein, M., Nassar, N. & Leberman, R. A second class of synthetase structure revealed by X-ray analysis of Escherichia coli seryl-tRNA synthetase at 2.5 Å. Nature 347, 249–255 (1990).
Rodin, S. N. & Ohno, S. Two types of aminoacyl-trna synthetases could be originally encoded by complementary strands of the same nucleic ACID. Origins Life Evol Biosphere 25, 565–589 (1995).
Carter, C. W. & Duax, W. L. Did tRNA Synthetase Classes Arise on Opposite Strands of the Same Gene? Molecular Cell 10, 705–708 (2002).
LéJohn, H. B., Cameron, L. E., Yang, B. & Rennie, S. L. Molecular characterization of an NAD-specific glutamate dehydrogenase gene inducible by L-glutamine. Antisense gene pair arrangement with L-glutamine-inducible heat shock 70-like protein gene. J Biol Chem 269, 4523–4531 (1994).
Carter, C. W. et al. The Rodin-Ohno hypothesis that two enzyme superfamilies descended from one ancestral gene: an unlikely scenario for the origins of translation that will not be dismissed. Biol Direct 9, 11 (2014).
Li, L., Francklyn, C. & Carter, C. W. Aminoacylating Urzymes Challenge the RNA World Hypothesis. Journal of Biological Chemistry 288, 26856–26863 (2013).
Chandrasekaran, S. N., Yardimci, G. G., Erdogan, O., Roach, J. & Carter, C. W. Statistical Evaluation of the Rodin–Ohno Hypothesis: Sense/Antisense Coding of Ancestral Class I and II Aminoacyl-tRNA Synthetases. Molecular Biology and Evolution 30, 1588–1604 (2013).
Pham, Y. et al. A Minimal TrpRS Catalytic Domain Supports Sense/Antisense Ancestry of Class I and II Aminoacyl-tRNA Synthetases. Molecular Cell 25, 851–862 (2007).
Martinez-Rodriguez, L. et al. Functional Class I and II Amino Acid-activating Enzymes Can Be Coded by Opposite Strands of the Same Gene. Journal of Biological Chemistry 290, 19710–19725 (2015).
Carter, C. W. An Alternative to the RNA World. Nat Hist 125, 28–33 (2016).
Rodin, S. N. & Rodin, A. S. Partitioning of Aminoacyl-tRNA Synthetases in Two Classes Could Have Been Encoded in a Strand-Symmetric RNA World. DNA and Cell Biology 25, 617–626 (2006).
Carter, C. What RNA World? Why a Peptide/RNA Partnership Merits Renewed Experimental Attention. Life 5, 294–320 (2015).
Ribas De Pouplana, L. & Schimmel, P. Two Classes of tRNA Synthetases Suggested by Sterically Compatible Dockings on tRNA Acceptor Stem. Cell 104, 191–193 (2001).
Carter, C. W. Coding of Class I and II Aminoacyl-tRNA Synthetases. in Protein Reviews (ed. Atassi, M. Z.) vol. 966 103–148 (Springer Singapore, Singapore, 2017).